![]() |
|
(575) 646-3801
Education
- Ph.D. Chemical Engineering, University of Virginia, 2000
- M.E. Chemical Engineering, University of Virginia, 1998
- B.S. Chemical Engineering, Iowa State University, 1990
Specialization
- Heterogeneous Catalysis
- Materials Characterization
- Renewable Fuels
Our work was featured on the cover of Catalysis Science & Technology:
Click here to see the journal.
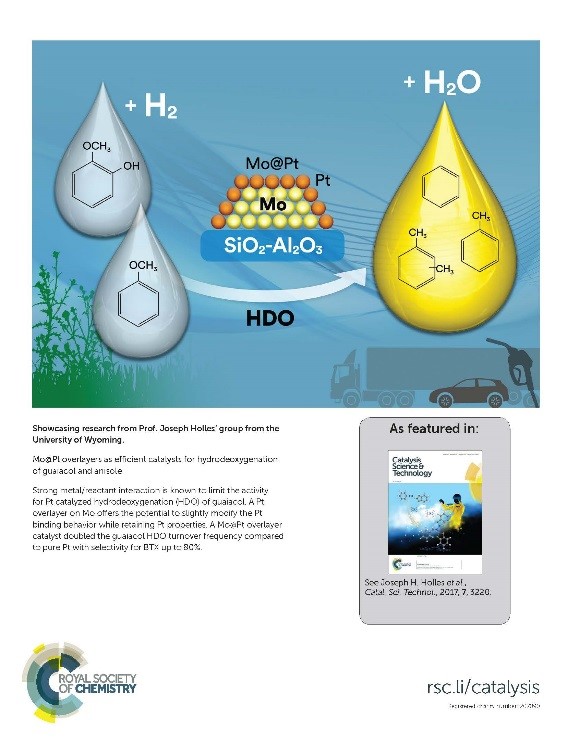
My laboratory research interest is nanoscale materials design and synthesis for catalytic applications. The design and synthesis is then complemented with reactivity studies and in-situ characterization of the catalytic material. In particular, X-ray absorption spectroscopy (XAS) experiments are periodically conducted at the Advanced Photon Source at Argonne National Laboratory. The combination of design, synthesis, and characterization allows for the direct translation of variations in the material at the atomic/molecular scale to expressed macroscopic properties such as improved reactivity. Our main area of current research involving nanoscale design and synthesis of catalytic materials is pseudomorphic overlayer bimetallic catalysts.
I am also interested in mentoring students, both undergraduate and graduate, in preparation for research to better prepare the students for research and to improve the research production. This includes developing and teaching undergraduate and graduate “theory and methods of research” type courses along with co-developing and co-teaching a Research Data Management course.
Pseudomorphic Overlayer Catalysts
Supported metal catalysts have been the mainstay of heterogeneous catalysts for many years. These catalysts are widely used since they combine the desirable catalytic properties of the metal with the enhanced activity resulting from their support on a high surface area material. More complicated reactions often use bimetallic catalysts. The drawback of bimetallic catalysts is that they are often a simple alloy resulting from an equilibrated mixture of the two metals. In particular, the bimetallic catalysts usually have both elements randomly distributed throughout the particle which therefore contains no atomic uniformity in the arrangement of the two elements. Design of supported bimetallic catalysts by synthesizing non-equilibrated arrangements could lead to new compositions of matter and ultimately to more efficient catalysts.
The importance of materials design at the atomic level is demonstrated through the use of computational quantum-chemical techniques. It has been shown theoretically that pseudomorphic overlayers of a metal on top of a different metal may provide lower intrinsic barriers to reaction products than pure metals [Figure 1]. Therefore, it is desirable to extend this concept from the theoretical to the practical by synthesizing actual supported metal catalysts that could have industrial applications. Our goal is to identify reactions where specific binding issues are known to affect reaction rates. We will then use overlayer catalysts to address these binding issues and increase reaction rates.
![]() |
|
Figure 1. Change in adsorption strength for overlayer metals on underlying host. |
We have examined core@shell Ni@Pt and Co@Pt catalysts for biorenewable fuel applications. We have looked at aqueous phase reforming (APR) of lactose to synthesis gas and aqueous phase hydrodeoxygenation (APH) of glycerol. In both cases, strong binding of CO is known to block catalyst sites and limit activity. Initial worked examined the ability for these overlayer catalysts to reduce the binding strength of the Pt overlayer metal. Figure 2 demonstrates how the hydrogen heat of adsorption for a Ni@Pt catalyst is decreased compared to pure Pt or a random structure Pt/Ni bimetallic catalyst.
![]() |
|
Figure 2. Hydrogen heat of adsorption is decreased for Ni@Pt overlayer catalyst compared to pure Pt or a random structure Ni/Pt bimetallic catalyst. |
These catalysts are then examined use a descriptor reaction. Ethylene hydrogenation is used as a descriptor reaction [Figure 3] since it is very sensitive to hydrogen surface coverage which is again strongly affected by hydrogen binding strength. For Ni@Pt, because the Ni host decreases the binding strength of the Pt, the hydrogen surface coverage should decrease and the reaction rate should decrease as observed in Figure 3. The catalysts have thus indicated that they may increase the activity of APR and APH reactions.
![]() |
|
Figure 3. Ethylene hydrogenation turnover frequency showing decreased activity (as a result of decreased hydrogen binding strength) for overlayer catalyst. |
Ni@Pt and Co@Pt catalysts were examined for APH of glycerol. In this reaction is desirable to remove the –OH functionalities (C-O bond breaking) of the molecule while minimizing C-C bond breaking. Figure 4 shows that Ni@Pt catalysts will increase the glycerol reaction rate compared to pure Pt. However, it results in a significant increase in C-C bond breaking as methane. In contrast, a Co@Pt catalyst not only increases the overall reaction rate, it produces similar amounts of the desired propane and propylene as the pure Pt.
![]() |
|
Figure 4. APH of glycerol for overlayer catalysts compared to pure Pt and random bimetallics. Overlayers conversion of glycerol compare to pure Pt. |
Similar APH results were also demonstrated for Ir@Pt overlayer catalysts. The Ir@Pt system was examined since Ir atoms have a similar size compared to Pt. The goal for this work was to determine if overlayer coverage could be increased when the two metals have more similar sizes. By using Ir as the base metal, we were able to double the surface Pt coverage compared to using a Ni base. Elemental mapping and elemental profiling in Figure 5 also shows that the Pt was well dispersed on the Pt in an overlayer type of structure.
![]() |
|
Figure 5. Elemental mapping and profiling of the Pt on Ir overlayer catalysts show a well dispersed Pt on Ir in an overlayer structure. |
If the core@shell catalysts are truly behaving in the desired manner, there should be a change in the electronics of the overlayer metal compared to a pure metal catalyst. When the binding strength is decreased, it is due to the underlying host metal withdrawing some electrons from the overlaying surface metal. This can be examined using X-ray Absorption Near Edge Spectroscopy (XANES) using the Advanced Photon Source at Argonne National Laboratory. Figure 6 shows the XANES spectra of a series of Ni@Pt overlayer, Pt-Ni bimetallic and pure Pt. The peak height of Ni@Pt SD (lowest Pt amount) is the highest. As more Pt is added, the Pt becomes more like pure Pt. Thus, the desired electronic effect has been demonstrated. While the Ni-Pt bimetallic shows a high Pt edge, extended x-ray absorption fine structure (EXAFS) analysis shows that it is Pt in a Ni matrix while the Ni@Pt shows Pt in an overlayer structure.
![]() |
|
Figure 6. XANES white line for Ni@Pt overlayer catalysts showing the electronic effect (higher edge height) for the overlayer catalysts compared to pure Pt. |
Using Life Cycle Assessment to Guide Catalysis Research
We have demonstrated the use of life cycle assessment (LCA) as a tool to drive catalyst development by comparing the environmental impact of acrylic acid production from propylene, the current commercial feedstock, to propane as an alternate feedstock. Acrylic acid is currently produced in a two step process from propylene. Because of its lower cost, propane is an attractive alternative to propylene; however no catalysts are currently available which can compete with the high yield of the propylene process. A comparison of the two feedstocks at the 87% yield of the current commercial propylene process demonstrated that switching to propane would decrease the environmental impact of the process by 20%. Determination of environmental impact as the yield from the potential propane process was varied, predicts that at yields exceeding 6%, the propane process will have a lower environmental impact than the current propylene process. The current catalyst yield of up to 48% for the propane process exceeds these values. If reaction and waste gas heat are converted to electricity instead of steam, yields in excess of 61% will result in a lower total impact for the propane process. Based on raw material costs, the economic break-even point for the propane process is 59% yield. The similar yields of ~60% from propane required by economics and for a lower environmental impact represents a factor of 1.25 increase in yield over the current state-of-the-art propane catalyst compared to a factor of 1.81 increase in yield required to equal the current propylene yield. Thus, the proposed propane process may be much closer to viability than previously realized. This analysis provides an example of how LCA can compare chemical production from two different feedstocks, even if a catalyst for the reaction of interest has not been designed. The LCA analysis can also be used to determine target goals for catalysis research.
Publications:
- Howe, L., and Holles, J.H., An Undergraduate Research Methods Class: Results and Experiences from Initial Offerings. Proceedings of the American Society for Engineering Education Annual Conference, Minneapolis MN (2022). https://peer.asee.org/an-undergraduate-research-methods-class-results-and-experiences-from-initial-offerings
- Holles, J.H., Initiating and Developing an Inclusive Physical Spaces Committee, Proceedings of the American Society for Engineering Education Annual Conference, Minneapolis MN (2022). https://peer.asee.org/initiating-and-developing-an-inclusive-physical-spaces-committee
- Holles, J.H., “Partnerships Between Preprofessional Student Groups and Your Unit,” Proceedings of the American Society for Engineering Education Annual Conference (2021). https://peer.asee.org/partnerships-between-preprofessional-student-groups-and-your-unit
- Holles, J.H., “Work in Progress: An Undergraduate Theory and Methods of Research Class for Honors Students,” Proceedings of the American Society for Engineering Education Annual Conference (2020). https://peer.asee.org/35611
- Holles, J.H., “Teaching Tip: Designing Your Course to Minimize Cheating” Chemical Engineering Education, 54 (2020) 42. https://journals.flvc.org/cee/article/view/114925/117050
- Holles, J.H., Work in Progress: Developing an Undergraduate Theory and Methods of Research Class for Honors Students, ASEE Rocky Mountain Section Conference, Laramie, WY, May 2019. https://www.uwyo.edu/seed/rms2019/papers/13_work_in_progress_developing_an_undergraduate_theory_and_methods_of_research_class_for_honors_students/13_work_in_progress_developing_an_undergraduate_theory_and_methods_of_research_class_for_honors_students.pdf
- Holles, J.H., Incorporating Research Data Management into an Existing Graduate Course on Theory and Methods of Research, ASEE Rocky Mountain Section Conference, Laramie, WY, May 2019. https://www.uwyo.edu/seed/rms2019/papers/14_incorporating_research_data_management_into_an_existing_graduate_course_on_theory_and_methods_of_research/14_incorporating_research_data_management_into_an_existing_graduate_course_on_theory_and_methods_of_research.pdf
- Holles, J.H., and Schmidt, L.O., Implementing a Graduate Class in Research Data Management for Science and Engineering Students, Paper ID #21190, Proceedings of the American Society for Engineering Education Annual Conference (2018). https://peer.asee.org/implementing-a-graduate-class-in-research-data-management-for-science-and-engineering-students
- Schmidt, L.O., and Holles, J.H., Teaching Research Data Management: It Takes a Team to Do It Right!, Paper ID #21194, Proceedings of the American Society for Engineering Education Annual Conference (2018). https://peer.asee.org/teaching-research-data-management-it-takes-a-team-to-do-it-right
- Holles, J.H., and Schmidt, L.O., Graduate Research Data Management Course Content: Teaching the Data Management Plan (DMP), Paper ID #21191, Proceedings of the American Society for Engineering Education Annual Conference (2018). https://peer.asee.org/graduate-research-data-management-course-content-teaching-the-data-management-plan-dmp
- Schmidt, L.O., and Holles, J.H., A Graduate Class in Research Data Management, Chemical Engineering Education, 52 (2018) 52.http://journals.fcla.edu/cee/article/view/105451/101127
- Lai, Q., Zhang, C., and Holles, J.H., “Mo@Pt Overlayers as Efficient Catalysts for Hydrodeoxygenation of Guaiacol and Anisole,” Catalysis Science & Technology, 7 (2017) 3220. http://pubs.rsc.org/en/content/articlepdf/2017/cy/c7cy00565b
- Zhang, C., Lai, Q., and Holles, J.H., “Bimetallic overlayer catalysts with high selectivity and reactivity for furfural hydrogenation,” Catalysis Communications, 89 (2017) 77. http://www.sciencedirect.com/science/article/pii/S1566736716303892
- Lai, Q., Skoglund, M.D., Zhang, C., Morris, A.R., and Holles, J.H., “Use of Hydrogen Chemisorption and Ethylene Hydrogenation as Predictors for Aqueous Phase Reforming of
Lactose over Ni@Pt and Co@Pt Bimetallic Overlayer Catalysts,” Energy and Fuels, 30 (2016) 8587. http://pubs.acs.org/doi/abs/10.1021/acs.energyfuels.6b01405 • Lai, Q., Zhang, C, and Holles, J.H., “Hydrodeoxygenation of Guaiacol over Ni@Pd and Ni@Pt Bimetallic Overlayer Catalysts,” Applied Catalysis A: General, 528 (2016) 1. http://www.sciencedirect.com/science/article/pii/S0926860X16304732 - Zhang, C., Lai, Q., and Holles, J.H., “Ir@Pt bimetallic overlayer catalysts for aqueous phase glycerol hydrodeoxygenation,” Applied Catalysis A: General, 526 (2016) 113. http://www.sciencedirect.com/science/article/pii/S0926860X16304185
- Zhang, C., Lai, Q., and Holles, J.H., Influence of Adsorption Strength in Aqueous Phase Glycerol Hydrodeoxygenation over Ni@Pt and Co@Pt Overlayer Catalysts” Catalysis Science and Technology 6 (2016) 4632. http://pubs.rsc.org/en/content/articlelanding/2016/cy/c6cy00097e#!divAbstract
- Morris, A.R., Skoglund, M.D., and Holles, J.H., Particle Size or Electronic Effect? An XAS Study of Re@Pd Overlayer Catalysts, Catal. Lett. 145 (2015) 840, http://link.springer.com/article/10.1007/s10562-015-1491-x
- Morris, A.R., Skoglund, M.D., and Holles, J.H., Characterization of Ni@Pt and Co@Pt overlayer catalysts using XAS studies, Applied Catalysis A, 489 (2015) 98, http://www.sciencedirect.com/science/article/pii/S0926860X14006322
- Huang, Z., Urynowicz, M.A., Holles, J.H., and Pribyl, R.D., Water Adsorption/Desorption Isotherms for Characterization of Micro porosity in Powder River Basin Subbituminous Coal, Int. J. Eng. Inv. Tech., 3 (12), (2014) 337. http://www.ijeit.com/Vol%203/Issue%2012/IJEIT1412201406_63.pdf
- Skoglund, M.D., Jackson, C., McKim, K., Olson, H.J., Sabirzyanov, S., and Holles, J,H. Characterization of Ni@Pt and Co@Pt Overlayer Catalysts Using Adsorption, Reactivity Descriptors, and X-ray Absorption Spectroscopy, Applied Catalysis A, 467 (2013) 355, http://www.sciencedirect.com/science/article/pii/S0926860X13004626#
- Skoglund, M.D., and Holles, J.H., Overlayer Bimetallic Catalysts or Particle Size Effects? A Comparison of Re@Pd Catalysts with Different Particle Sizes, Catalysis Letters, 143 (2013) 966, http://link.springer.com/article/10.1007%2Fs10562-013-1052-0
- Jackson, C., and Holles, J.H., Who is Who in Kinetics, Reaction Engineering, and Catalysis, Chemical Engineering Education, 47(4) (2013) 197.
- Bommineni, S., Skoglund, M.D., Morris, A., Doskocil, E.J., and Holles, J.H., Characterization of Selective Oxidation Catalysts from Polyoxometalate Precursors Using Ammonia Adsorption Microcalorimetry and Methanol Oxidation Studies, Applied Catalysis A, 467 (2013) 202, http://www.sciencedirect.com/science/article/pii/S0926860X13004298
- Holman, P.A., Shonnard, D.R., and Holles, J.H., Using Life Cycle Assessment to Guide Catalysis Research, Industrial and Engineering Chemistry Research, 48(14), 6668-6674 (2009). http://pubs.acs.org/doi/abs/10.1021/ie801934s
- Latusek, M.P., Spigarelli, B.P., Heimerl, R.M., and Holles, J.H., Correlation of H2 Heat of Adsorption and Ethylene Hydrogenation Activity for Supported Re@Pd Overlayer Catalysts, Journal of Catalysis, 263, 306-314 (2009). http://www.sciencedirect.com/science/article/pii/S0021951709000657
- Latusek, M.P., Heimerl, R.M., Spigarelli, B.P., and Holles, J.H., Synthesis and Characterization of Supported Bimetallic Overlayer Catalysts, Applied Catalysis A; General, 358, 79-87 (2009). [http://www.sciencedirect.com/science/article/pii/S0926860X09000969
- Holles, J.H., Old Dead Guys: Using Activity Breaks to Teach History, Chemical Engineering Education, 43(2), 1-3 (2009).
- Chia, Y.N., Latusek, M.P., and Holles, J.H., Catalytic Wet Oxidation of Lactose, Industrial & Engineering Chemistry Research, 47(12), 4049-4055 (2008). http://pubs.acs.org/doi/abs/10.1021/ie701779u
- Holles, J.H., A Graduate Course in Theory and Methods of Research, Chemical Engineering Education, 41(4), 226-232 (2007).
- Dillon, C.J., Holles, J.H., Davis, R.J., Labinger, J.A., and Davis, M.E., A Substrate-Versatile Catalyst for the Selective Oxidation of Light Alkanes: II. Catalyst Characterization. Journal of Catalysis, 218, 54-66 (2003). http://www.sciencedirect.com/science/article/pii/S0021951703000095
- Holles, J.H., Dillon, C.J., Labinger, J.A., and Davis, M.E., A Substrate-Versatile Catalyst for the Selective Oxidation of Light Alkanes: I. Reactivity. Journal of Catalysis, 218, 42-53 (2003). http://www.sciencedirect.com/science/article/pii/S0021951703000083
- Dillon, C.J., Holles, J.H., Davis, M.E., and Labinger, J.A., Heteropolyacid-based Catalysts for Selective Alkane Oxidation: Mechanism of Formation of Maleic Acid from Propane. Catalysis Today, 81, 189-195 (2003). http://www.sciencedirect.com/science/article/pii/S0920586103001123
- Davis, M.E., Dillon, C.J., Holles, J.H., and Labinger, J., A New Catalyst for the Selective Oxidation of Butane and Propane. Angewandte Chemie 114, 886-890 (2002). http://onlinelibrary.wiley.com/doi/10.1002/1521-3757(20020301)114:5%3C886::AID-ANGE886%3E3.0.CO;2-K/epdf
- Davis, M.E., Dillon, C.J., Holles, J.H., and Labinger, J., A New Catalyst for the Selective Oxidation of Butane and Propane. Angewandte Chemie Int. Ed. 41, 858-860 (2002). http://onlinelibrary.wiley.com/doi/10.1002/1521-3773(20020301)41:5%3C858::AID-ANIE858%3E3.0.CO;2-7/abstract
- Holles, J.H., and Davis, R.J., Structure of Pd/CeOx/Al2O3 Catalysts for NOx Reduction Determined By In Situ X-ray Absorption Spectroscopy. Journal of Physical Chemistry, B, 104, 9653-9660 (2000). http://pubs.acs.org/doi/abs/10.1021/jp001347r
- Holles, J.H., and Davis, R.J., Murray, T.M., and Howe, J.M., Effects of Pd Particle Size and Ceria Loading on NO Reduction with CO. Journal of Catalysis 195, 193-206 (2000). http://www.sciencedirect.com/science/article/pii/S0021951700929853
- Holles, J.H., Switzer, M.A., and Davis, R.J., Influence of Ceria and Lanthana Promoters on the Kinetics of NO and N2O Reduction by CO over Alumina Supported Palladium and Rhodium. Journal of Catalysis 190, 247-260 (2000). http://www.sciencedirect.com/science/article/pii/S002195179992780X
Patents:
- Holles, J.H., Catalytic Wet Oxidation of Lactose. U.S. Patent #7,371,362, May 13, 2008.
- Brait, A., Davis, M.E., Dillon, C.J., Holles, J.H., and Labinger, J., Polyoxometalate Catalysts and Catalytic Process. U.S. Patent #7,019,165, March 28, 2006.
- Brait, A., Davis, M.E., Dillon, C.J., Holles, J.H., and Labinger, J., "Polyoxometalate Catalysts and Catalytic Process." U.S. Patent #6,914,029, July 5, 2005.